A Closer Look: How Viruses Change Over Time
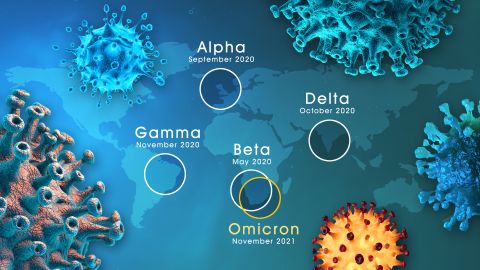
Every few months, the news seems to have a story about some new strain of SARS-CoV-2, the coronavirus that causes COVID-19. From Delta to Omicron and now beyond to Omicron’s numerous subvariants, SARS-CoV-2 has been changing ever since it first came to our attention in late 2019. But where do these viral strains and variants come from? When should they be concerning? How do viral changes influence ongoing vaccination efforts? In this issue, we’ll cover some of the basics regarding how viruses mutate and evolve over time, how this can affect us, and how we can adapt to combat these changes.
Why do viruses change?
Viruses, just like us, have genomes, or blueprints, that dictate their characteristics, replication and survival. While plants, animals, and many microbes (including bacteria and some viruses), use DNA to store these genomes, some viruses (SARS-CoV-2 among them) use RNA, a molecule similar to DNA.
RNA and DNA are known as “nucleic acids,” and they are made up of long strings of individual “nucleotides,” which act almost like letters in an alphabet. This is why you might see DNA sequences with the letters A, C, G, and T in different orders. The sequence of letters dictates what proteins are made. When a virus replicates, its progeny virions will have the same exact genome encoding the same exact proteins – almost. Why almost? Because mistakes can happen during this replication process. Let’s take a closer look at how these mistakes occur and what consequences can arise.
When an organism duplicates its genome during cell division, errors can happen during the process. While there are systems in place within the cell that proofread new copies of the genome, no system is perfect. Occasionally, the new copy of the genome bears a mistake akin to a biological typo. We refer to these biological typos as “mutations.” A lot of the time, mutations have no effect on the ability of the organism to function normally. Sometimes, mutations can be detrimental, and rarely, mutations can be helpful, causing the production of a protein that functions even better than the older version and improving the organism’s overall fitness or ability to reproduce.
In humans, some detrimental mutations are well-known, such as those that can cause cancers or genetic disorders. Beneficial mutations, on the other hand, occur so rarely that we generally only realize they’ve occurred after generations. On the other hand, viruses replicate so often that mutations can be realized much more quickly.
How do mutations lead to new strains?
For a virus, the difference between a detrimental and a beneficial mutation is often quite simple. For example, a detrimental mutation would be one that causes the virus to spread and reproduce less, while a beneficial mutation would enable the virus to spread and reproduce more.
When a person is infected with a virus, that single infection can sometimes lead to more than 100 billion new viral particles within one person. This volume of viral production provides ample opportunity for new mutations to arise. New viral particles that carry beneficial mutations will be more likely to survive and generate offspring of their own causing those virions to become more and more common in the population. This aspect of viral evolution is described as natural selection. Eventually, these more successful offspring viruses, which accrue more mutations along the way, will be so different from their ancestor virus that they’re classified as a new strain of the virus. Virologists and epidemiologists who study viruses monitor these changes and work to understand how the strains differ – not only genetically, but also in terms of their ability to infect people, spread, or cause harm.
How do new strains affect people?
When a person is infected with a virus like SARS-CoV-2, our body responds by making antibodies that target certain parts of the virus, known as “antigens.” The SARS-CoV-2 virus has several antigens, including the spike protein which gives the virus its recognizable spiked ball shape. The adaptive immune system learns to recognize these antigens so that it can react quickly should it encounter that virus again. This is also the principle upon which vaccines operate.
Vaccines teach the adaptive immune system to efficiently recognize a new pathogen and mount an immune response against it to prepare for a future encounter. However, the battle between humans and viruses is ongoing, and a virus with mutations that evade existing human immunity will be more likely to thrive than a virus that the immune system recognizes. This happens because the antibodies bind to virus particles they recognize, and thus viruses with mutations that can evade detection will go on to infect cells and reproduce.
Importantly, while all viruses mutate, the ability to accumulate mutations conducive to survival is variable. For example, DNA viruses, like hepatitis B or varicella (chickenpox), tend to be more stable than RNA viruses, like measles or human immunodeficiency virus (HIV), due to differences between the two nucleic acids (DNA and RNA). Indeed, studies have shown that RNA viruses can evolve up to one million times faster than DNA viruses. Likewise, different RNA viruses have developed in ways that rely on various genetic and structural protections for survival. For example, the structure of measles virus protects the surface location for binding to cells during an infection, such that mutations are unlikely to accumulate in this region. Conversely, HIV mutations accumulate at such a rate that an infection within a single individual consists of multiple forms of HIV virus, making it difficult for the individual’s immune system to overcome the infection. These different approaches to RNA virus survival mean that vaccines for some of them, like measles, are easier to develop and maintain than others, such as HIV for which we have not yet successfully developed a vaccine.
How has SARS-CoV-2 changed since it arose?
Scientific evidence has shown us that the SARS-CoV-2 virus jumped from an animal to a person or people at a live animal market in the Hubei province of China in late 2019. Much like when new strains emerge in people, this initial “jump” was likely the result of an accumulation of mutations that allowed the virus to reproduce better in people than in the animal it was already infecting. These mutations then led to a new type of human infection that, for some, was deadly.
From this initial event, SARS-CoV-2 continued to reproduce and, therefore, mutate, making it better equipped to infect people and resulting in multiple waves of new strains. To better track and report how the virus changed, the WHO assigned names based on the Greek alphabet to the most widespread strains. The Alpha and Beta strains were variants of concern near the end of 2020, until the Delta strain emerged and outcompeted them. Delta was then the dominant strain for about 12 months, at which point the now-infamous Omicron strain exploded onto the scene and took over. In 2023, we continue to wrestle with the many subvariants of Omicron.
With each new strain of SARS-CoV-2, we have witnessed subtle changes in disease. For example, the Delta variant was more likely to cause unvaccinated patients to be hospitalized. Omicron, meanwhile, appears to spread more readily than previous strains, but seems to cause less severe disease when contracted. It’s hard to know for certain because while SARS-CoV-2 has changed, so, too, have the people it infects. Since the virus was first encountered, people have been developing avoidance strategies, gaining natural immunity, and getting vaccinated. Scientists around the world are continuing to monitor the changes in SARS-CoV-2, so that as the virus changes, we can be prepared.
Related resources
How Do Viruses Reproduce? (animation)
The Adaptive Immune System (animation)
How the Immune System Works (webpage)
Omicron, Delta, Alpha, and More: What To Know About the Coronavirus Variants (article)